Sustainability Assessment of the HIGFLY Process: Advancing Sustainable Aviation Fuel Production
The HIGFLY project aims to develop advanced technologies for producing sustainable aviation fuels (SAF) using hemicellulose C5 sugar streams from lignocellulosic biorefineries. Researchers from ifeu have evaluated the sustainability performance of the HIGFLY process through environmental, economic, and social assessments.
The environmental assessment uses life cycle assessment (LCA) methodology to compare the impacts of different scenarios, while the economic assessment applies life cycle costing (LCC) methodology. The social assessment uses the social life cycle assessment (sLCA) and the Social Hotspot Database (SHDB) to identify potential social risks and benefits.
Environmental impacts
The environmental impact of the HIGFLY process is calculated using the internationally standardised life cycle assessment (LCA) methodology, which follows the principles outlined in ISO 14040:2006 and ISO 14044:2006. The LCA methodology involves four iterative phases: goal and scope definition, inventory analysis, impact assessment, and interpretation.
- Goal and Scope Definition: This phase sets the objectives of the LCA and defines the system boundaries, functional unit, and reference system. For the HIGFLY project, the goal is to assess the sustainability performance of producing sustainable aviation fuels (SAF) from lignocellulosic biomass. The system boundaries include the entire life cycle from biomass cultivation to the production and use of SAF components. The functional unit is defined as 1 megajoule (MJ) of fuel, ensuring comparability with conventional jet fuel.
- Inventory Analysis: This phase involves collecting data on all inputs and outputs associated with the HIGFLY process. Foreground data, such as mass and energy balances, are provided by the project consortium, while background data, such as upstream raw material and energy supply chains, are sourced from databases like Ecoinvent. The inventory includes data on biomass cultivation, biorefinery processes, solvent use, energy consumption, and emissions.
- Impact Assessment: In this phase, the collected inventory data are translated into potential environmental impacts using characterisation models. The HIGFLY LCA considers various impact categories, including climate change (GHG emissions), freshwater ecotoxicity, freshwater eutrophication, terrestrial acidification, and non-renewable energy resource depletion. Each impact category is assessed using specific indicators and characterisation models, such as the Global Warming Potential (GWP) for climate change.
- Interpretation: The final phase involves analysing the results to identify key drivers of environmental impacts and potential areas for improvement. Sensitivity analyses are conducted to understand the influence of different assumptions and methodological choices, such as allocation methods and the use of alternative solvents or biorefinery technologies.
The LCA results for the HIGFLY process reveal a heterogeneous picture of environmental performance. While some scenarios show significant GHG savings compared to fossil jet fuel, other impact categories, such as freshwater and terrestrial acidification, may exhibit higher emissions. The assessment identifies the use of fossil-based solvents and acetone, as well as the energy source for the biorefinery, as major contributors to environmental impacts. Increasing process efficiencies and using green energy sources are recommended to further improve the environmental performance of the HIGFLY process.
Economic assessment
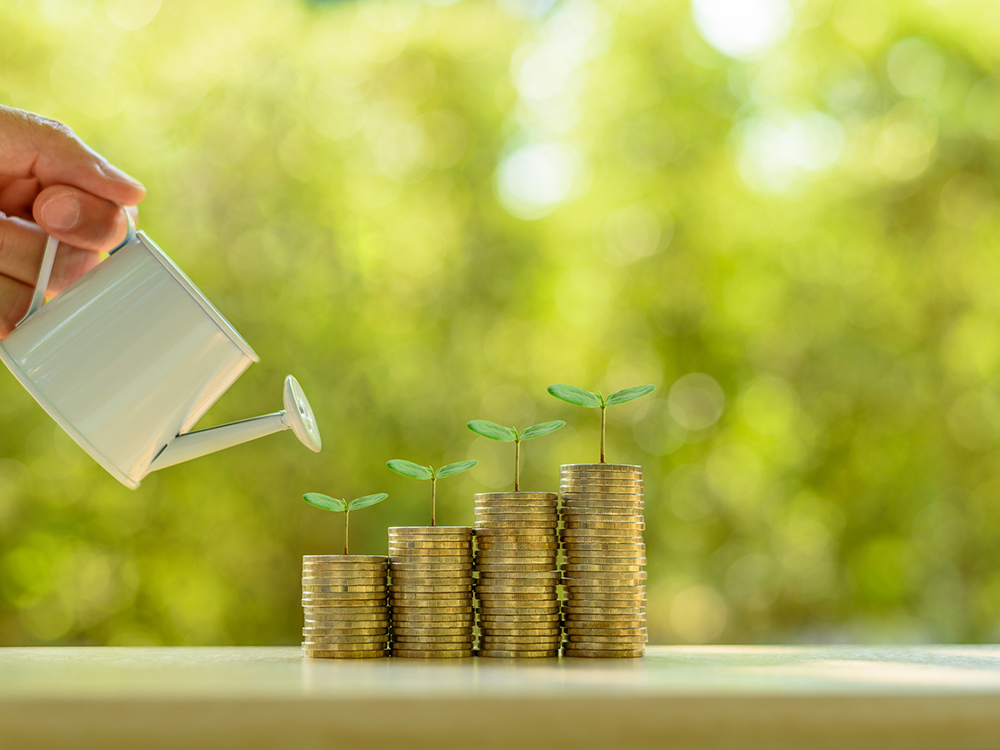
The economic assessment of the HIGFLY process is conducted using the life cycle costing (LCC) methodology, which records all costs occurring along the life cycle of a product. The LCC methodology is laid out in the UNEP/SETAC guidelines for life cycle costing.
The LCC assessment for the HIGFLY process captures all costs from feedstock acquisition to sustainable aviation fuel (SAF) production, aiming to optimise budget allocation, identify cost drivers, and highlight areas for cost reduction. The analysis covers the entire process chain with a chosen base Scenario. Variations are included to account for different production sites and market price fluctuations.
Data is categorised into site-dependent costs, such as electricity, water, labour, and heating (wood chips), and site-independent costs, like acetone, solvents, and hydrogen. Prices are varied to reflect low, average, and high-cost scenarios, with location-based costs modelled for potential plant sites in the Netherlands, France, and Romania. Global market prices inform site-independent costs. CAPEX and OPEX estimates account for price uncertainties.
The Levelised Cost of Fuel (LCOF) is calculated per tonne of SAF, including costs for C5 sugar, electricity, heat, solvents, acetone, hydrogen, water, CAPEX, labour, and OPEX. Various scenarios evaluate cost and efficiency impacts on the LCOF. A sensitivity analysis examines side stream valorisation by producing internal hydrogen through aqueous phase reforming (APR), using literature data to assess its influence on LCOF.
The assessment reveals C5 sugar as the main cost driver, followed by CAPEX, labour, hydrogen, and other operational expenses. Minimum cost scenarios show that LCOF could be significantly lower than the current HEFA market price. Though APR has limited impact on cost reduction, results underscore the importance of choosing an optimal production site and the potential effects of future market prices on the HIGFLY process´ economic viability and that further cost reductions can be achieved through efficiency gains and favourable market conditions.
By conducting this detailed economic assessment, the HIGFLY project identifies key cost drivers and potential areas for cost optimisation, providing valuable insights for future development and commercialisation of sustainable aviation fuel technologies.
Social impacts
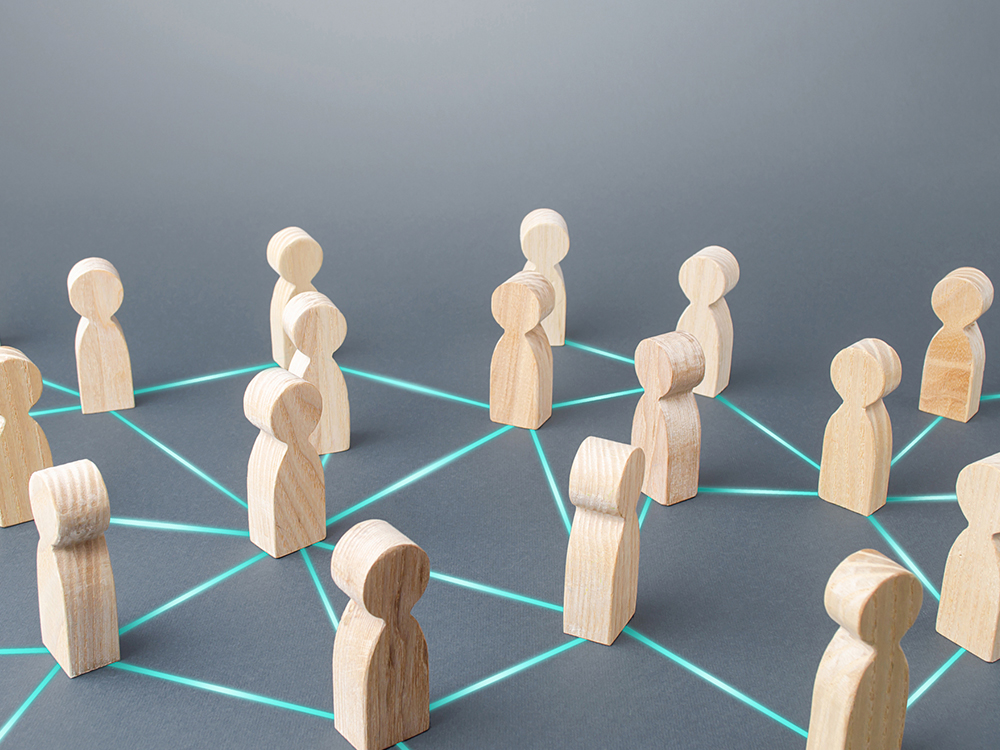
The social impacts of the HIGFLY process are evaluated using Social Life Cycle Assessment (sLCA) methodology, which identifies effects on the well-being of those involved. This assessment follows established sLCA guidelines and uses the Reference Scale Assessment (RS) method to classify social risks, such as 'low', 'medium', or 'high'. The Social Hotspot Database (SHDB) supports this analysis by categorising risks for each social indicator based on standards, local laws, and best practices.
The sLCA spans the entire production chain, from feedstock sourcing to sustainable aviation fuel (SAF) production, using a “cradle-to-gate” scope that captures all processes up to fuel output. A Scenario is chosen as the baseline, with additional scenarios varying efficiency levels and input sources. Data on social risks are primarily drawn from the SHDB, aligned with the Global Trade
Analysis Project (GTAP) model, while production costs and input/output data come from economic assessments.
The geographical scope includes 57 sectors across 144 countries, allowing inputs to be mapped to specific countries and sectors. For instance, the baseline assumes Dutch inputs, while sensitivity analyses consider Romanian inputs. Chemical and hydrogen imports are modelled from countries like China and Morocco. Social risks are calculated by multiplying workhours for each input by the country- and sector-specific social risk factors, producing results in medium-risk work-hour equivalents across categories such as 'Labour Rights and Decent Work', 'Health and Safety', and 'Governance'.
The analysis reveals varied social risks across supply chain stages, with chemicals and hydrogen provision posing the highest risks due to high-risk origin countries. Biorefinery operations have relatively lower risks. Results also show that factors like efficiency improvements and sourcing from different countries can significantly influence social risks.
The sLCA identifies potential social benefits as well, including job creation, economic value, and rural development. The use of agricultural residues for biomass feedstock enhances food security by avoiding competition with food production. Implementing the HIGFLY process can support quality employment and economic growth, especially in rural areas.
In conclusion, social risks in the HIGFLY process mainly arise from imported chemicals and hydrogen, particularly when sourced from high-risk regions. European production site choices have minimal impact on social risks but may increase social benefits. By pinpointing these risks, HIGFLY provides insights for responsible sourcing and sustainable practices, paving the way for a more socially responsible SAF production.
Overall assessment
Overall, the HIGFLY project demonstrates the potential for producing sustainable aviation fuels with lower environmental impacts, competitive costs, and manageable social risks. The findings highlight the need for further research and development to optimise the process and integrate biorefinery concepts. The project also underscores the importance of clear and consistent regulatory frameworks to support the adoption of novel technologies and ensure long-term planning security for economic operators.
The aim of this research is to prove that the HIGFLY technologies and processes, and those like it, can contribute to the European Union´s broader goals of climate change mitigation, economic prosperity, and social resilience. And it is hoped that the results of the sustainability assessments provide valuable insights for policymakers, researchers, and industry stakeholders to guide future developments and investments in sustainable aviation fuels and the production of bio-chemicals.